- What is Uranium. Uranium is a naturally-occurring chemical element with atomic number 92 which means there are 92 protons and 92 electrons in the atomic structure.The chemical symbol for uranium is U.Uranium was discovered in 1789 by Martin Klaproth in the mineral called pitchblende (uraninite). He named the newly discovered element after the planet Uranus, which had been discovered eight.
- Atomic number The number of protons in an atom. Electron configuration The arrangements of electrons above the last (closed shell) noble gas. Uranium-235 is the only naturally occurring fissionable fuel (a fuel that can sustain a chain reaction). Uranium fuel used in nuclear reactors is enriched with uranium-235.
- Element Uranium (U), Group 20, Atomic Number 92, f-block, Mass 238.029. Sources, facts, uses, scarcity (SRI), podcasts, alchemical symbols, videos and images.
Uranium is a common naturally occurring and radioactive substance. It is a normal part of rocks, soil, air, and water, and it occurs in nature in the form of minerals – but never as a metal. Uranium metal is silver-colored with a gray surface and is nearly as strong as steel.Natural uranium is a mixture of three types or isotopes called U-234/234U, U-235/235U and U-238/238U. Since the German chemist Martin Heinrich Klaproth identified uranium in 1789, atomic number 92 has become one of the most troubling substances on the planet.
- Nuclear properties
- Processes of nuclear decay
- Nuclear structure and shape
- Extension of the periodic table
- Transactinoid elements and their predicted properties
Our editors will review what you’ve submitted and determine whether to revise the article.
Join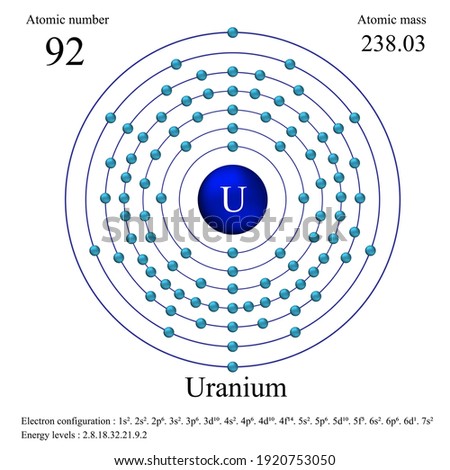
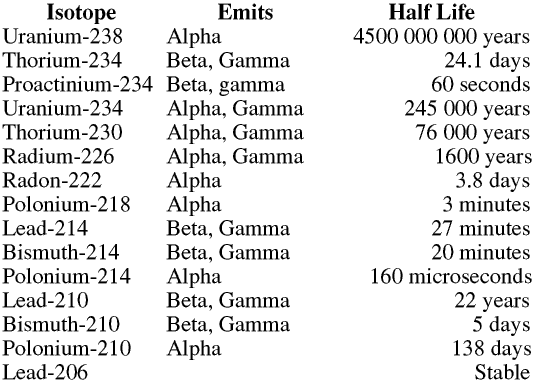
Transuranium element, any of the chemical elements that lie beyond uranium in the periodic table—i.e., those with atomic numbers greater than 92. Twenty-six of these elements have been discovered and named or are awaiting confirmation of their discovery. Eleven of them, from neptunium through lawrencium, belong to the actinoid series. The others, which have atomic numbers higher than 103, are referred to as the transactinoids. All the transuranium elements are unstable, decaying radioactively, with half-lives that range from tens of millions of years to mere fractions of a second.
Since only two of the transuranium elements have been found in nature (neptunium and plutonium) and those only in trace amounts, the synthesis of these elements through nuclear reactions has been an important source of knowledge about them. That knowledge has expanded scientific understanding of the fundamental structure of matter and makes it possible to predict the existence and basic properties of elements much heavier than any currently known. Present theory suggests that the maximum atomic number could be found to lie somewhere between 170 and 210, if nuclear instability would not preclude the existence of such elements. All these still-unknown elements are included in the transuranium group.
Discovery of the first transuranium elements
The first attempt to prepare a transuranium element was made in 1934 in Rome, where a team of Italian physicists headed by Enrico Fermi and Emilio Segrè bombarded uranium nuclei with free neutrons. Although transuranium species may have been produced, the experiment resulted in the discovery of nuclear fission rather than new elements. (The German scientists Otto Hahn, Fritz Strassman, and Lise Meitner showed that the products Fermi found were lighter, known elements formed by the splitting, or fission, of uranium.) Not until 1940 was a transuranium element first positively produced and identified, when two American physicists, Edwin Mattison McMillan and Philip Hauge Abelson, working at the University of California at Berkeley, exposed uranium oxide to neutrons from a cyclotron target. Mosfet 6426. One of the resulting products was an element found to have an atomic number of 93. It was named neptunium.
Uranium Atomic Number Periodic Table
Transformations in atomic nuclei are represented by equations that balance all the particles of matter and the energy involved before and after the reaction. The above transformation of uranium into neptunium may be written as follows:
In the first equation the atomic symbol of the particular isotope reacted upon, in this case U for uranium, is given with its mass number at upper left and its atomic number at lower left: 23892U. The uranium-238 isotope reacts with a neutron (symbolized n, with its mass number 1 at upper left and its neutral electrical charge shown as 0 at lower left) to produce uranium-239 (23992U) and the quantum of energy called a gamma ray (γ). In the next equation the arrow represents a spontaneous loss of a negative beta particle (symbolized β−), an electron with very high velocity, from the nucleus of uranium-239. What has happened is that a neutron within the nucleus has been transformed into a proton, with the emission of a beta particle that carries off a single negative charge; the resulting nucleus now has one more positive charge than it had before the event and thus has an atomic number of 93. Because the beta particle has negligible mass, the mass number of the nucleus has not changed, however, and is still 239. The nucleus resulting from these events is an isotope of the element neptunium, atomic number 93 and mass number 239. The above process is called negative beta-particle decay. A nucleus may also emit a positron, or positive electron, thus changing a proton into a neutron and reducing the positive charge by one (but without changing the mass number); this process is called positive beta-particle decay. In another type of beta decay a nuclear proton is transformed into a neutron when the nucleus, instead of emitting a beta particle, “captures,” or absorbs, one of the electrons orbiting the nucleus; this process of electron capture (EC decay) is preferred over positron emission in transuranium nuclei.
The discovery of the next element after neptunium followed rapidly. In 1941 three American chemists, Glenn T. Seaborg, Joseph W. Kennedy, and Arthur C. Wahl, produced and chemically identified element 94, named plutonium (Pu). In 1944, after further discoveries, Seaborg hypothesized that a new series of elements called the actinoid series, akin to the lanthanoid series (elements 58–71), was being produced, and that this new series began with thorium (Th), atomic number 90. Thereafter, discoveries were sought, and made, in accordance with this hypothesis.
Synthesis of transuranium elements
The most abundant isotope of neptunium is neptunium-237. Neptunium-237 has a half-life of 2.1 × 106 years and decays by the emission of alpha particles. (Alpha particles are composed of two neutrons and two protons and are actually the very stable nucleus of helium.) Neptunium-237 is formed in kilogram quantities as a by-product of the large-scale production of plutonium in nuclear reactors. This isotope is synthesized from the reactor fuel uranium-235 by the reaction
Iwork 09 high sierra. and from uranium-238 by
Plutonium, as the isotope plutonium-239, is produced in ton quantities in nuclear reactors by the sequence
Because of its ability to undergo fission with neutrons of all energies, plutonium-239 has considerable practical applications as an energy source in nuclear weapons and as fuel in nuclear power reactors.
The method of element production discussed thus far has been that of successive neutron capture resulting from the continuous intensive irradiation with slow (low-energy) neutrons of an actinoid target. The sequence of nuclides that can be synthesized in nuclear reactors by this process is shown in the figure, in which the light line indicates the principal path of neutron capture (horizontal arrows) and negative beta-particle decay (up arrows) that results in successively heavier elements and higher atomic numbers. (Down arrows represent electron-capture decay.) The heavier lines show subsidiary paths that augment the major path. The major path terminates at fermium-257, because the short half-life of the next fermium isotope (fermium-258)—for radioactive decay by spontaneous fission (370 microseconds)—precludes its production and the production of isotopes of elements beyond fermium by this means.
Heavy isotopes of some transuranium elements are also produced in nuclear explosions. Typically, in such events, a uranium target is bombarded by a high number of fast (high-energy) neutrons for a small fraction of a second, a process known as rapid-neutron capture, or the r-process (in contrast to the slow-neutron capture, or s-process, described above). Underground detonations of nuclear explosive devices during the late 1960s resulted in the production of significant quantities of einsteinium and fermium isotopes, which were separated from rock debris by mining techniques and chemical processing. Again, the heaviest isotope found was that of fermium-257.
An important method of synthesizing transuranium isotopes is by bombarding heavy element targets not with neutrons but with light charged particles (such as the helium nuclei mentioned above as alpha particles) from accelerators. For the synthesis of elements heavier than mendelevium, so-called heavy ions (with atomic number greater than 2 and mass number greater than 5) have been used for the projectile nuclei. Targets and projectiles relatively rich in neutrons are required so that the resulting nuclei will have sufficiently high neutron numbers; too low a neutron number renders the nucleus extremely unstable and unobservable because of its resultantly short half-life.
Uranium Atomic Number And Mass
The elements from seaborgium to copernicium have been synthesized and identified (i.e., discovered) by the use of “cold,” or “soft,” fusion reactions. In this type of reaction, medium-weight projectiles are fused to target nuclei with protons numbering close to 82 and neutrons numbering about 126—i.e., near the doubly “magic” lead-208—resulting in a relatively “cold” compound system. The elements from 113 to 118 were made using “hot” fusion reactions, similar to those described above using alpha particles, in which a relatively light projectile collides with a heavier actinoid. Because the compound nuclei formed in cold fusion have lower excitation energies than those produced in hot fusion, they may emit only one or two neutrons and thus have a much higher probability of remaining intact instead of undergoing the competing prompt fission reaction. (Nuclei formed in hot fusion have higher excitation energy and emit three to five neutrons.) Cold fusion reactions were first recognized as a method for the synthesis of heavy elements by Yuri Oganessian of the Joint Institute for Nuclear Research at Dubna in the U.S.S.R. (now in Russia).
- key people
- related topics
